The progress made in atom manipulation over the last two decades has allowed us to use the quantum properties of matter to make accurate inertial sensors.
The best sensitivity in absolute gravimetry comes from quantum sensors, which join the clocks for accurate measurements given by quantum instruments. Other types of quantum sensors make highly accurate measurements such as rotation, acceleration, magnetic field, or light, as shown in Figure 1.

Quantum instruments are built according to the recipe: a sensor head, where the atoms are kept in an ultra-high vacuum environment to be interrogated; magnetic field environment, made of coils and magnetic shields to control the magnetic field applied on the atoms; a laser system, to cool and manipulate the atoms through the different steps; a microwave chain, to generate the frequencies required for the laser system; and a controller, to define the sequence and collect the data.
Use quantum gravity gradiometers to measure density fluctuations
Gravity gradiometers are used to map the underground density anomaly by measuring the gradient of gravity, which gives the same information as gravity with less signal at long distances but more sensitivity to density fluctuations.
To do so, the vertical acceleration of two different clouds of cold atoms is measured simultaneously with the same interrogation laser, as represented in Figure 2. That allows the suppression of common-mode noise between the two clouds, which allows gradiometers to perform measurements in noisy environments such as onboard vehicles.
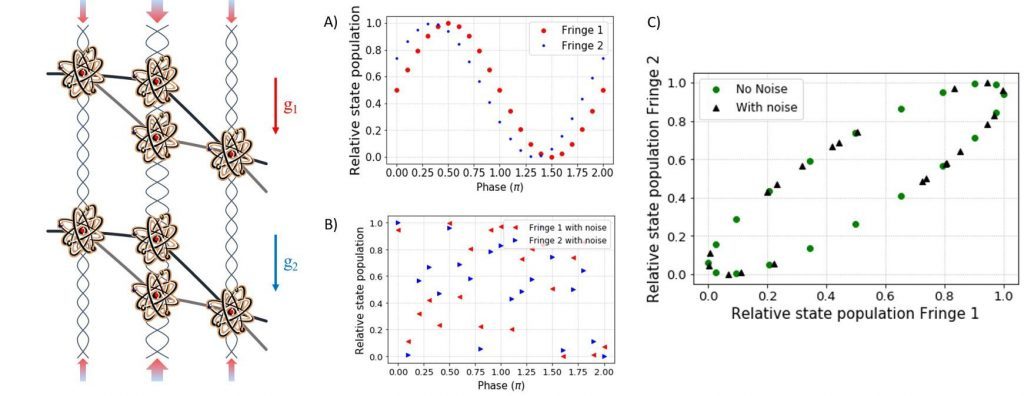
The quantum gravity gradiometer is the preferred choice for industrial transfer because quantum instruments demonstrate high performance and common-mode suppressed gradiometers can perform measurements in different environments.
The University of Birmingham leads the UK Quantum Technology Hub for Sensors and Timing and developed 79 collaborative projects with the industry, corresponding to a GBP 62 million project value with 132 invention records. One of the major goals is to develop compact field quantum gravity gradiometers with a technology readiness suitable for industrial development.
Atom interferometry requires fast frequency modulation, a narrow linewidth, and low noise
Our quantum gravity gradiometer is based on cooled Rubidium-87 atoms. A cloud consisting of 108 atoms is cooled and trapped by laser beams. The cloud is cooled through different steps, first via magneto-optical trapping, then by performing optical molasses to reach 4 μK. To cool the atoms, the laser frequency is red-detuned from the atomic transition.
For interrogation, vertically aligned laser beams put the atoms into a quantum superposition state. Then, after an evolution time, they are recombined by laser pulses (of a few μs).
The output state of the interferometer, read by fluorescence, depends on the ability of the laser frequency chirp to compensate for the changing Doppler shift induced by the acceleration of the atoms in freefall.
As the laser frequency must be kept stable, the laser seed Koheras BASIK E15 from NKT Photonics is locked to a rubidium transition using a spectroscopy cell. The laser intensity, the laser linewidth, and the laser frequency directly impact the interferometer phase, so the laser system needs to be as good as possible in order not to add extra noise.
Our instrument requires a fast frequency modulation (100 MHz in 1 ms), a narrow linewidth (1 kHz), a low phase noise, and a low relative intensity noise. Moreover, the polarisation extinction ratio must be high, as the atomic transition efficiency depends on the polarisation.
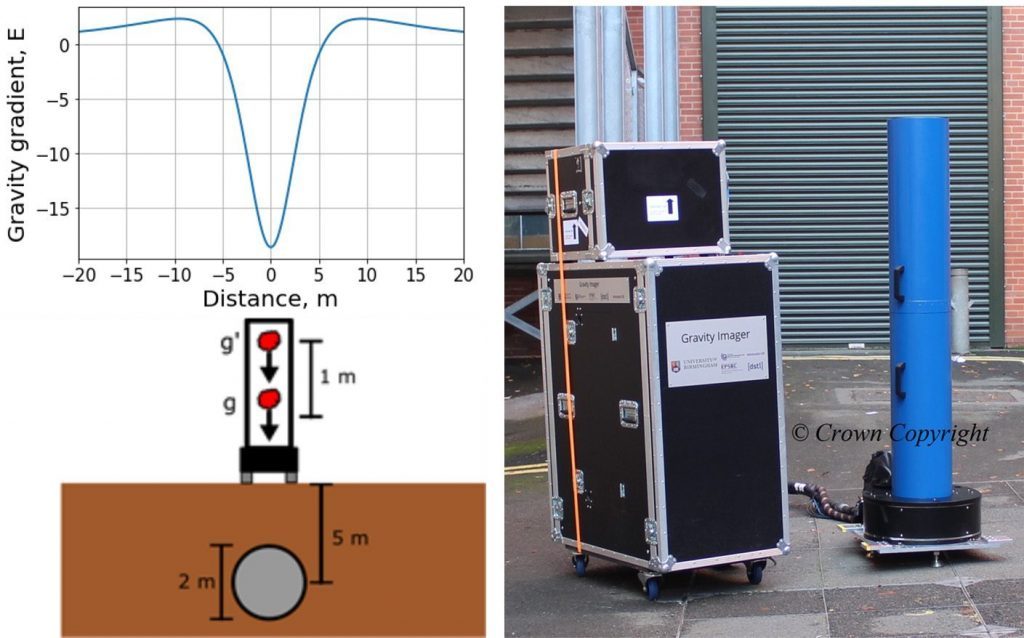
Our laser systems are mainly based on fiber frequency-doubled telecom technology, which allows for a compact, robust, and transportable instrument. The laser frequency is shifted or modified by an EOM (electro-optic modulator), or an IQM (in-phase/quadrature modulator).
The optical power is amplified by up to 2 W by a Koheras BOOSTIK amplifier module, the light is switched on and off by an AOM (acousto-optic modulator) and, finally, the wavelength is converted from 1560 nm to 780 nm by a second harmonic generator.
At the University of Birmingham, we are also using more recent cold atoms tools, such as moving lattices. We are investigating how best to interrogate atoms with a large momentum transfer, e.g. using the high-power frequency-doubled laser system Koheras HARMONIK.
Compact systems enable more applications
The current field gradiometer from the University of Birmingham is the world’s most portable quantum gravity gradiometer, designed to detect under-surface tunnels as illustrated in Figure 3.
This instrument shows a useful application for civil engineering for non-destructive underground mapping. It is already in the industrial transfer process with the UK industry, via the Innovate UK-funded grant Gravity Pioneer.
To investigate the potential for application areas such as navigation, we are working on making the systems more compact. We investigate how we can build smaller sensor heads, and we test magneto-optical trapping on drones, as shown in Figure 4.
Next, we will explore how to implement new methods to reach higher sensitivity in more compact schemes.

Learn more about quantum gravity gradiometers
References
Clemens Rammeloo, Lingxiao Zhu, Yu-Hung Lien, Kai Bongs, and Michael Holynski, “Performance of an optical single-sideband laser system for atom interferometry“, J. Opt. Soc. Am. B 37, 1485-1493 (2020).
Bongs, K., Holynski, M., Vovrosh, J. et al., “Taking atom interferometric quantum sensors from the laboratory to real-world applications”, Nat Rev Phys 1, 731–739 (2019).
Lingxiao Zhu, Yu-Hung Lien, Andrew Hinton, Alexander Niggebaum, Clemens Rammeloo, Kai Bongs, and Michael Holynski, “Application of optical single-sideband laser in Raman atom interferometry“, Opt. Express 26, 6542-6553 (2018).
Gradiometer team members
Luuk Earl, Farzad Hayati, Sam Hedges, Andrew Lamb, Mehdi Langlois, David Sedlak, Ben Stray, Hester Thomas, Jamie Vovrosh, Jonathan Winch led by Kai Bongs and Michael Holynski.
For project information contact
Mehdi Langlois
Quantum Technology Hub Sensors and Timing
Midland Ultracold Atom Research Centre
School of Physics and Astronomy
University of Birmingham
m.langlois[at]bham.ac.uk