One advantage of perovskites is that they can be easily manufactured from common salts in low-temperature processes – such as spin coating – and can be made into ink and printed onto flexible substrates to form flexible solar cells. Another advantage is that perovskites can be designed to absorb the wavelengths of the sun more efficiently than silicon.
Perovskites are a vast number of compounds having a perovskite crystal structure. The general chemical formula is ABX3, where A and B are cations and X is an anion.
Nevertheless, a lot of research is needed in perovskite photovoltaics e.g. to improve their scaling, efficiency, stability, lifetime, and how to passivate defects to increase efficiency. Today, solar cells made from crystalline silicon boast efficiencies above 25% where. Perovskite solar cells are already above 20%, up from 13% in 2012.
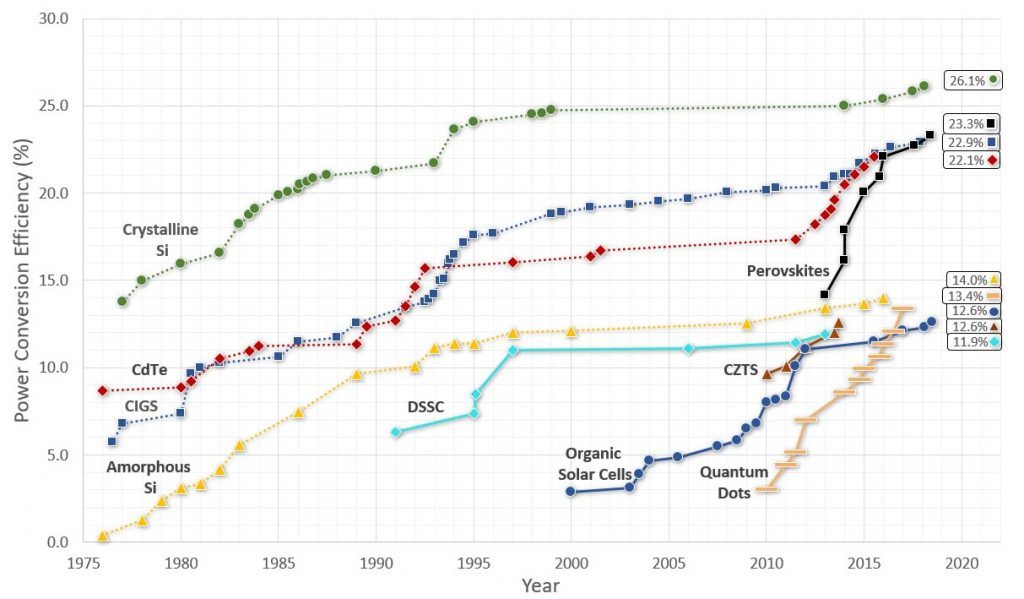
Lasers are already routinely used to investigate how light interacts with perovskites, spectrally and dynamically, on the micrometer scale. Compared to traditional light sources, lasers allow for the in-depth analysis that is needed to continue the flow of break-throughs in perovskite research. One of today’s challenges is to find the optimal composition of A, B, and X. The hunt is on…!
Efficiency mapping using a broad spectrum supercontinuum laser
It is critical to know how efficiently the perovskite crystals can absorb the different wavelengths of the solar spectrum. Such measurements can be carried out through photocurrent spectroscopy and imaging.
Our SuperK white light laser and SuperK VARIA filter offer the broad spectral coverage needed to investigate materials across the solar spectrum. The SuperK provides picosecond pulsed operation and a high-quality beam, allowing investigations of the optical properties with a diffraction-limited resolution. A set-up measuring absorption coefficient and refractive index can be seen in Fig. 4.
Understanding the structural properties with a picosecond pulse source
Structural properties, such as sample uniformity and defects, can help understand the limitations of the material. Here, hyperspectral imaging and exciton diffusion mapping can come in handy. The spectrum and picosecond pulse property of the SuperK white light laser supports the simultaneous acquisition of spectral and temporal data.
The unique properties of the SuperK laser allow you to have a picosecond pulse source at any wavelength in the visible and near-infrared spectrum. Combined with single-photon detection this allows for mapping of exciton lifetimes and diffusion lengths on a micrometer scale, unveiling exciton dynamics and defect densities only achievable through the versatility of the SuperK technology.
Example of perovskite researchers who have used our SuperK lasers and tunable filters in their set-ups
Professor Urban’s Nanospectroscopy Group, Ludwig-Maximilians University
Professor Urban’s group used the set-up in figure 2 to measure exciton lifetime.
The combination of a SuperK laser, a spectrometer, and a fast single-photon detector (an APD) made it possible to obtain spectral and lifetime information. High power enabled faster acquisition from the highly absorbing material.
A short cut-in wavelength was mandatory to get as close to the standard solar spectrum (e.g. ASTM G-173-03) as possible, making our SuperK FIU-15 or EXU-6 obvious choices.
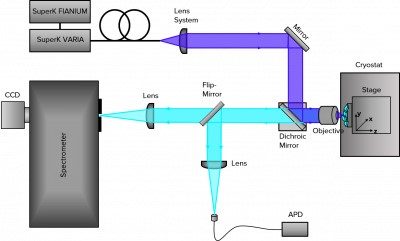
Professor Urban’s group added cameras to the set-up in figure 2 to enable measurements of exciton diffusion lengths, see figure 3.
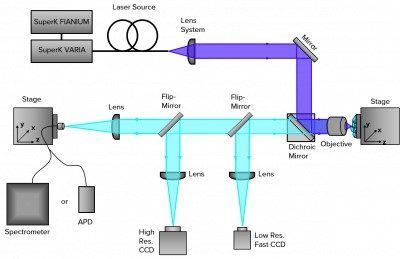
Sarah Brittman and Erik C. Garnett, Center for Nanophotonics, FOM Institute AMOLF
Sarah Brittman and Erik C. Garnett were performing refractive index and absorption coefficient imaging. Light from a supercontinuum laser was filtered by an acousto-optic tunable filter (the SuperK SELECT AOTF) and focused by an objective onto the sample, which was mounted on a piezostage.
Three photodetectors measured the incident, transmitted, and reflected beams at each wavelength produced by scanning the AOTF. A half-waveplate was used to control the laser’s polarization. A flip mirror, lens, and camera were used to image the sample to locate the crystals.
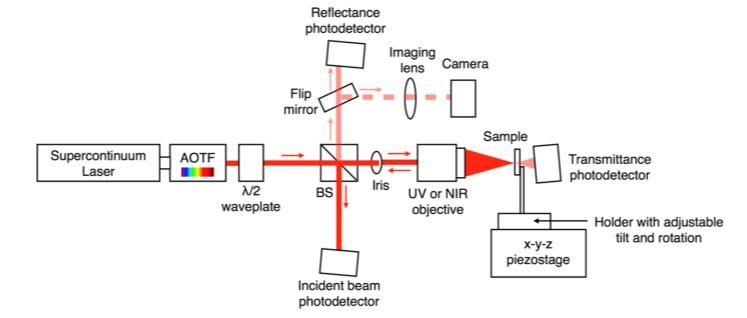
The SuperK enables the following measurements
- Pump-probe spectroscopy
- Refractive index
- Absorption coefficient imaging
- Photoluminescence
- Emission (exciton) lifetime; multidimensional
- Quantum efficiency (IPCE vs QE); solar simulation
- Single-photon counting
Papers describing perovskite research using our SuperK white light laser.
- Photoprotection in metal halide perovskites by ionic defect formation by Nga Phung, Alessandro Mattoni, Joel A.Smith, Dieter Skroblin, Hans Köbler, Leo Choubrac, Joachim Breternitz, Jinzhao Li, Thomas Unold, Susan Schorr, Christian Gollwitzer, Ivan G. Scheblykin, Eva L. Unger, Michael Saliba, Simone Meloni, Antonio Abate, Aboma Merdasa published in Joule, 2022.
- Self-assembly, stability, and photoresponse of PbS quantum dot films capped with mixed halide perovskite ligands by Samaneh Aynehband, Maryam Mohammadi, Rana Poushimin, Mahdi Hasanzadeh Azar, Jean-Michel Nunzi, Abdolreza Simchi published in Materials Research Bulletin, 2022, DOI: 10.1016/j.materresbull.2021.111648.
- Controllable Acceleration and Deceleration of Charge Carrier Transport in Metal-Halide Perovskite Single-Crystal by Cs-Cation Induced Bandgap Engineering by Mehri Ghasemi, Yurou Zhang, Chunhua Zhou, Cheng Tan, Eunyoung Choi, Jae Sung Yun, Aijun Du, Jung-Ho Yun, Baohua Jia, Xiaoming Wen published in small, 2022. DOI: 10.1002/smll.202107680.
- Probing the effect of a glass network on the synthesis and luminescence properties of composite perovskite glasses by Anna Karagiannaki, Ioannis Konidakis, George Kourmoulakis, Ioanna Demeridou, Jana Dzibelova, Aristides Bakandritsos, Emmanuel Stratakis published in Optical Materials Express, 2022. DOI: 10.1364/OME.445927.
- The Structural Origin of Chiroptical Properties in Perovskite Nanocrystals with Chiral Organic Ligands by Young-Hoon Kim, Ruyi Song, Ji Hao, Yaxin Zhai, Liang Yan, Taylor Moot, Axel F. Palmstrom, Roman Brunecky, Wei You, Joseph J. Berry, Jeffrey L. Blackburn, Matthew C. Beard, Volker Blum, Joseph M. Luther published in Advanced Functional Materials, 2022. DOI: 10.1002/adfm.202200454.
- Electron-Hole Binding Governs Carrier Transport in Halide Perovskite Nanocrystal Thin Films by Michael F. Lichtenegger, Jan Drewniok, Andreas Bornschlegl, Carola Lampe, Andreas Singldinger, Nina A. Henke, Alexander S. Urban published in Materials Science, 2022, DOI:10.48550/arXiv.2201.12473.
- Revealing the doping density in perovskite solar cells and its impact on device performance by Francisco Peña-Camargo, Jarla Thiesbrummel, Hannes Hempel, Artem Musiienko, Vincent M. Le Corre, Jonas Di published in Materials Science, 2022, DOI: 10.48550/arXiv.2201.09664.
- Understanding the Effect of Lead Iodide Excess on the Performance of Methylammonium Lead Iodide Perovskite Solar Cells by Zeeshan Ahmad, Rebecca A. Scheidt, Matthew P. Hautzinger, Kai Zhu, Matthew C. Beard, Giulia Galli published in Materials Science, 2022, DOI:10.48550/arXiv.2201.12473.
- Dark and Bright Excitons in Halide Perovskite Nanoplatelets by Moritz Gramlich, Michael W. Swift, Carola Lampe, John L. Lyons, Markus Döblinger, Alexander L. Efros, Peter C. Sercel, Alexander S. Urban published in Advanced Science, 2021, DOI: 10.1002/advs.202103013.
- Resonantly Pumped Bright-Triplet Exciton Lasing in Cesium Lead Bromide Perovskites by Guanhua Ying, Tristan Farrow, Atanu Jana, Hanbo Shao, Hyunsik Im, Vitaly Osokin, Seung Bin Baek, Mutibah Alanazi, Sanjit Karmakar, Manas Mukherjee, Youngsin Park, Robert A. Taylor published in ACS Photonics, 2021, DOI: 10.1021/acsphotonics.1c00720.
- Fully Vacuum-Processed Perovskite Solar Cells on Pyramidal Microtextures by Lidón Gil-Escrig, Marcel Roß, Johannes Sutter, Amran Al-Ashouri, Christiane Becker, Steve Albrecht published in RRL Solar, 2021, DOI: 10.1002/solr.202000553.
- Development of colloidal quantum dot and lead halide perovskite light emitting devices thesis by Sihan Xie, Ph.D., Massachusetts Institute of Technology, 2021.
- Minimising Optical and Electrical Losses in Perovskite Solar Cells − From Single-Junctions to Advanced Tandem Designs thesis by M.Sc. Philipp Tockhorn, University of Berlin, 2021.
- Interdependence of photon upconversion performance and antisolvent processing in thin-film halide perovskite-sensitized triplet–triplet annihilators by Karunanantharajah Prashanthan, Boris Naydenov, Klaus Lips, Eva Unger, Rowan W. MacQueen published in the Journal of Chemical Physics, 2020, DOI: 10.1063/5.0026564.
- CsI-Antisolvent Adduct Formation in All-Inorganic Metal Halide Perovskites by Taylor Moot, Ashley R. Marshall, Lance M. Wheeler, Severin N. Habisreutinger, Tracy H. Schloemer, Caleb C. Boyd, Desislava R. Dikova, Gregory F. Pach, Abhijit Hazarika, Michael D. McGehee, Henry J. Snaith, Joseph M. Luther published in Advanced Energy Materials, 2020, DOI: 10.1002/aenm.201903365.
- Large lattice distortions and size-dependent bandgap modulation in epitaxial halide perovskite nanowires by Eitan Oksenberg, Aboma Merdasa, Lothar Houben, Ifat Kaplan-Ashiri, Amnon Rothman, Ivan G. Scheblykin, Eva L. Unger, Ernesto Joselevich published in Nature Communications, 2020, DOI: 10.1038/s41467-020-14365-2.
- Humidity-robust scalable metal halide perovskite film deposition for photovoltaic applications by Carlo Andrea Riccardo Perini, Anil Reddy Pininti, Samuele Martani, Peter Topolovsek, Andrea Perego, Daniele Cortecchia, Annamaria Petrozza, Mario Caironi published in Journal of Materials Chemistry A, 2020, DOI: 10.1039/d0ta05003b.
- Tuning the Structural and Optoelectronic Properties of Cs2AgBiBr6 Double-Perovskite Single Crystals through Alkali-Metal Substitution by Masoumeh Keshavarz, Elke Debroye, Martin Ottesen, Cristina Martin, Heng Zhang, Eduard Fron, Robert Küchler, Julian A. Steele, Martin Bremholm, Joris Van de Vondel, Hai I. Wang, Mischa Bonn, Maarten B. J. Roeffaers, Steffen Wiedmann, Johan Hofken published in Advanced Materials, 2020, DOI: 10.1002/adma.202001878.
- Crucial role of charge transporting layers on ion migration in perovskite solar cells by Abasi Abudulimua, Lang Liub, Guilin Liuc, Nijiati Aimaiti, Bohuslav Rezeka, Qi Chen published in Journal of Energy Chemistry, 2020, DOI: 10.1016/j.jechem.2019.12.002
- Controlling competing photochemical reactions stabilizes perovskite solar cells by Silvia G. Motti, Daniele Meggiolaro, Alex J. Barker, Edoardo Mosconi, Carlo Andrea Riccardo Perini, James M. Ball, Marina Gandini, Min Kim, Filippo De Angelis, Annamaria Petrozza published in Nature Photonics, 2019.
- Efficient perovskite solar cells by hybrid perovskites incorporated with heterovalent neodymium cations by Kai Wang, Luyao Zheng, Tao Zhu, Xiang Yao, Chao Yi, Xiaotao Zhang, Yu Cao, Lei Liu, Wenping Hu, Xiong Gong published in Nano Energy, 2019.
- Phase Behavior and Substitution Limit of Mixed Cesium-Formamidinium Lead Tri-Iodide Perovskites by Bethan Charles, Mark T. Weller, Sebastian Rieger, Lauren E. Hatcher, Paul F. Henry, Jochen Feldmann, Daniel Wolverson, Chick C. Wilson published in Chemistry of Materials, 2020, DOI: 10.1021/acs.chemmater.9b04032
- Nonradiative Energy Transfer between Thickness-Controlled Halide Perovskite Nanoplatelets by Andreas Singldinger, Moritz Gramlich, Christoph Gruber, Carola Lampe, and Alexander S. Urban published in ACS Energy Letters April 2020, 5. DOI: 10.1021/acsenergylett.0c00471.
- Polymer Nanoreactors Shield Perovskite Nanocrystals from Degradation by Verena A. Hintermayr, Carola Lampe, Maximilian Löw, Janina Roemer, Willem Vanderlinden, Moritz Gramlich, Anton X. Böhm, Cornelia Sattler, Bert Nickel, Theobald Lohmüller, and Alexander S. Urban, published in Nano Letters 2019 19 (8), 4928-4933. DOI: 10.1021/acs.nanolett.9b00982.
- Impact of Excess Lead Iodide on the Recombination Kinetics in Metal Halide Perovskites by Aboma Merdasa, Alexander Kiligaridis, Carolin Rehermann, Mojtaba Abdi-Jalebi, Jonas Stöber, Boris Louis, Marina Gerhard, Samuel D. Stranks, Eva L. Unger, and Ivan G. Scheblykin, published in ACS Energy Letters 2019 4 (6), 1370-1378. DOI: 10.1021/acsenergylett.9b00774.
- Measuring n and k at the Microscale in Single Crystals of CH3NH3PbBr3 Perovskite by Sarah Brittman and Erik C. Garnett, published in The Journal of Physical Chemistry C 2016 120 (1), 616-620. DOI: 10.1021/acs.jpcc.5b11075.
- Highly luminescent cesium lead halide perovskite nanocrystals with tunable composition and thickness by ultrasonication by Yu Tong, Eva Bladt, Meltem F. Aygüler, Aurora Manzi, Dr. Karolina Z. Milowska, Verena A. Hintermayr, Prof. Dr. Pable Docampo, Prof. Dr. Sara Bals, Dr. Alexander S. Urban, Dr. Lakshminarayana Polavarapu, and Prof. Dr. Jochen Feldmann, published in Angewandte Chemie International Edition 2016 55.44, 2016. DOI: 10.1002/anie.201605909.