Accurate quantum inertial sensors
If you can track your rotation and acceleration in any direction over time, you can calculate your exact position and velocity relative to your starting point – without relying on external input such as GPS or landmarks.
The accuracy of your inertial sensor determines the accuracy of your position and velocity derived from the measured acceleration. Today, the most accurate inertial sensors are based on atom interferometry which is created by cooling and manipulating individual (typically alkaline) atoms with laser beams.
A popular choice of atom for atom interferometry is Rubidium-87 which requires a high power 780 nm beam of light with narrow linewidth and high beam quality.
You need accurate lasers to cool atoms
Laser cooling is a critical step that requires a tunable narrow-linewidth high-power laser such as our Koheras BASIK. The rubidium ions are typically cooled in a magneto-optical trap, MOT. A MOT uses a combination of magnetic fields and a narrow-linewidth high-power laser at 780 nm to cool and trap the ions to place them in an appropriate quantum state for further investigation by, e.g., atom interferometry.
Atom interferometry
Atom interferometry uses the wave property of atoms to place an atom in a superposition between two states: One state where the atom has been “hit” by a light pulse, and one where it was not. Something that will send the two states along different paths.
Just as light, these two states can undergo interference if brought back together. Any perturbation along their paths, such as the forces from rotational or translational acceleration will affect how the matter-wave interference pattern turns out.
Pick a stable laser
The Koheras DFB fiber lasers are well known for their unmatched Hz-level linewidth at watt-power levels. But DFB fiber lasers only come in a few infrared wavelength bands. And these bands rarely match the wavelengths needed for laser cooling of atoms and ions.
Frequency conversion gives you 780 nm
We suggest seeding an Erbium-Doped Fiber Amplifier (EDFA), such as the Koheras BOOSTIK HP, with a stable narrow linewidth seed laser such as the Koheras BASIK at 1560 nm. Use the Koheras HARMONIK frequency conversion module to efficiently convert the high-power light at 1560 nm into high-power light at 780 nm.
You get a 780 nm fiber laser system at watt level with a beam quality M2 ≈ 1.1 and a free-running linewidth less than 1 kHz – the ideal laser system for any Rb atom interferometer.
Frequency conversion is a nonlinear process. The HARMONIK frequency conversion module only converts the main laser signal while cleaning up any unwanted power at out-of-band wavelengths present in most lasers.
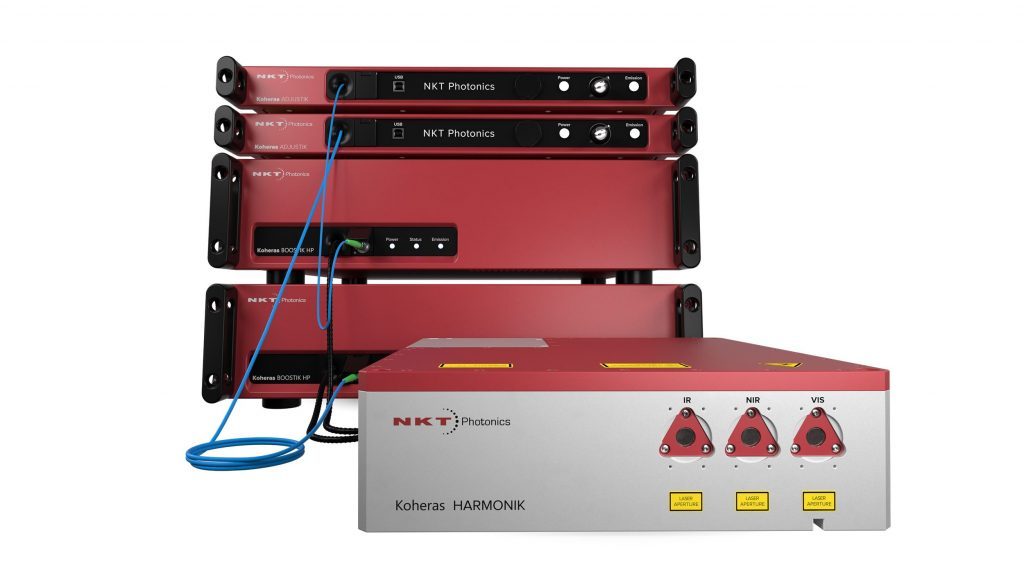
Robust enough for oil rigs and sophisticated enough for the lab
Our fiber laser design is inherently compact and robust. It is developed for a lifetime of above 10 years in demanding environments where uptime is critical. With failure rates lower than 1%, we proudly deliver the most reliable low-noise lasers on the market. Alignment-free and maintenance-free.
The industrial-grade OEM lasers have a rugged design, a stable performance unaffected by changing environmental conditions, and wide temperature ranges in the field as well as the lab. We deliver lasers to the most advanced laboratories worldwide such as the Institute of Quantum Optics at Leibniz University Hannover and the UK Quantum Technology Hub for Sensors and Timing at the University of Birmingham. We have more than 15,000 Koheras lasers deployed in the harshest environments on – and off – the planet. We have lasers on oil rigs, submarines, wind turbines, and even in space. With over 20 years of experience, we know they last. Also in your lab.
References
- Time-domain optics for atomic quantum matter by Simon Kanthak, Martina Gebbe, Matthias Gersemann, Sven Abend, Ernst M. Rasel, Markus Krutzik published in New Journal of Physics, 2021.
- Twin-lattice atom interferometry by Martina Gebbe, Jan-Niclas Siemss, Matthias Gersemann, Hauke Müntinga, Sven Herrmann, Claus Lämmerzahl, Holger Ahlers, Naceur Gaaloul, Christian Schubert, Klemens Hammerer, Sven Abend, and Ernst M. Rasel published in Nature Communications, 2021.
- Optical frequency generation using fiber Bragg grating filters for applications in portable quantum sensing by Calum D. Macrae, Kai Bongs, and Michael Holynski published in Optics Letters, 46, 2021.
- A 40 W, 780 nm laser system with compensated dual beam splitters for atom interferometry by Minejeong Kim, Remy Notermans, Chris Overstreet, Joseph Curti, Peter Asenbaum, Mark A. Kasevich published in Optics Letters, 2020.
- Doppler Compensated Cavity For Atom Interferometry by Rustin Nourshargh , Sam Hedges, Mehdi Langlois, Kai Bongs, and Michael Holynski in Atomic Physics, 2020.
- Performance of an optical single-sideband laser system for atom interferometry by Clemens Rammeloo, Lingxiao Zhu, Yu-Hung Lien, Kai Bongs, and Michael Holynski published in Journal of the Optical Society of America B, 2020.
- Taking atom interferometric quantum sensors from the laboratory to real-world applications by Kai Bongs, Michael Holynski, Jamie Vovrosh, Philippe Bouyer, Gabriel Condon, Ernst Rasel, Christian Schubert, Wolfgang P. Schleich, and Albert Roura published in Nature Reviews Physics, 2019.
- A portable magneto-optical trap with prospects for atom interferometry in civil engineering by A. Hinton, M. Perea-Ortiz, J. Winch, J. Briggs, S. Freer, D. Moustoukas, S. Powell-Gill, C. Squire, A. Lamb, C. Rammeloo, B. Stray, G. Voulazeris, L. Zhu, A. Kaushik, Y.-H. Lien, A. Niggebaum, A. Rodgers, A. Stabrawa, D. Boddice, S. R. Plant, G. W. Tuckwell, K. Bongs, N. Metje, and M. Holynski published in Philosophical transactions of the Royal Society A, 2017.
Our quantum engagements
We are part of the European Quantum Flagship, the European Quantum Industry Consortium, the Quantum Economic Development Consortium, and the Danish Quantum Community.